By Jason Palidwar, Claude Montcalm, and Peter Hook, Iridian Spectral Technologies
Wildfires are a growing problem in North America and across the world as extreme weather events like drought become both more severe and more prolonged. Across the U.S., 64,897 wildfires were reported in 2024, destroying more than 4,500 structures and consuming nearly 9 million acres of land — more than triple the acreage destroyed by wildfires in the U.S. in 2023.1 And these statistics do not include the California wildfires that, since January 2025, have been responsible for 29 deaths, more than 150,000 people displaced, and 16,000+ structures destroyed.2
Saving lives, land, and property hinges heavily on early discovery of fires that often start in remote areas and remain undetected until they have grown catastrophically, as well as early recognition of the conditions that can lead to wildfires. Fire lookout towers have been used for this purpose for decades, supplemented, in some cases, by high-altitude observational aircraft. However, advances in optical technology, combined with changing launch cost dynamics, have pushed optical Earth observation (EO) to the forefront of wildfire detection, tracking, and management.
Earth Observation Enters its Age of Viability
Until about the last decade, Earth observation was primarily the realm of space agencies, since EO satellites were large and expensive to build and launch. But two key changes have taken place. First, the advent of CubeSats and other pint-sized satellites, such as Planet’s Dove satellites, has significantly reduced the weight and size of EO platforms, making it more physically and financially feasible to launch those units as “rideshares” on space-bound craft.
Second, the emergence of commercial launch programs such as SpaceX and Rocket Lab has resulted in a massive increase in the number of launches — from just a few a year to hundreds3 — dramatically increasing opportunities for launch and drastically reducing the cost-per-kilogram-per-launch to send equipment into space.4 Additionally, these launches often offer a “ride-share” service where as many as 100+ satellites from different organizations share the payload space.5
This accessibility has exponentially increased interest in and accessibility to EO in the commercial sector, including in fire prevention. Examples of such systems include FireSat, a first-phase constellation is slated for launch in 2026, and OroraTech, which currently operates two EO satellites and has plans to launch 100+ similar satellites.
What Are You Looking For?
The application of EO to early remote detection of wildfires typically offers two key approaches. First, observers can attempt to identify chemical absorption bands of interest, such as potassium, that can be indicative as precursors to wildfire. More common is the use of thermal imaging to look for hot spots, or specific types of heat profile, in longwave infrared (LWIR) bands from space. Multispectral imaging systems can combine these approaches into one unit. There is an advantage to comparing signals from different wavelength bands, or emission lines, to distinguish between “normal” situations and undesirable ones, as well as to confirm the presence of a wildfire.
To achieve multiband detection, one can place a multizone filter on top of a detector, in close proximity, to where each zone of the filter will transmit a particular wavelength region onto the detector surface. For example, a multizone optical filter may have several strips of filters split between wavelength regions in the visible spectrum, as well as mid-wave infrared (MWIR) and LWIR bands. Each strip, or zone, usually translates into a different gas/element/constituent being detected. However, the detector is a fixed area, so the more zones in that area, the narrower the band, and the fewer pixels assigned to each one — which can limit the signal relative to the background for each band.
Thus, designers must navigate the tradeoffs between what they want to detect and any limitations imposed by the detector itself. Dropping a noncritical band or two from the design can improve signal quality for the other bands as well as help to optimize cost for the satellite creator/operator.
How Iridian Spectral Technologies Can Help
Initial interactions with a customer provide an opportunity for us to discuss the many specification trade-offs, such as having steep edges/deep blocking versus the cost of the filter, which can inform the customer’s decision on how many bands to include. There is a tendency to want to include everything possible at first, but in addition to size, weight, and power (SWaP) considerations that are part of any optical system being sent into space, signal quality is impacted by band count. Multizone optical filters (or arrays) contain dead zones (between each zone): pixels blocked out due to the physical seams or transition zones between the systems’ functional bands (Fig. 1). More signal zones/active pixels equal more dead zones/blocked pixels, which can quickly become untenable when attempting to pack more and more spectral bands into a single element.
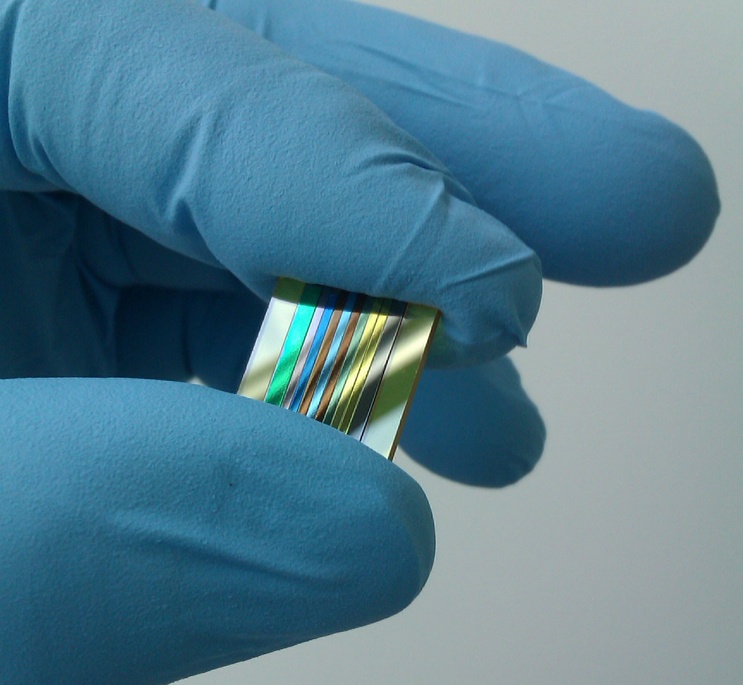
Fig. 1 — A multizone optical filter array. Note the dark seams (“dead zones”) between each of the array’s functional bands.
Often, multiple parties are involved in this collaboration. Iridian Spectral Technologies’ direct interaction usually is with an integrator or an optical designer at the camera/imaging company, but the end user/customer is the organization creating the satellite(s) that will ultimately use the data generated by those optics. Multi-party interactions are invaluable toward clear communication of designs and intent. They also save time (and, by extension, cost) versus long, iterative back-and-forths.
This is particularly important relevant to the angle of incidence and the optical system’s focusing properties. For example, a customer system featuring a span of angles will impact how the optical filters perform because the band pass will not be as square. Finalizing the angles created by other optics in the design before considering filters limits what can be achieved using those filters. Angle or design tweaks may be necessary to achieve the desired system performance. So, to arrive at the best technical and commercial solution, it is valuable for us to not only be in contact with the integrator or optical designer but also with the end user as early as possible.
When a customer approaches us with a description/specifications for the spectral filtration they require, one of our first steps after navigating the trade-offs above is to provide a design curve, i.e., a spectral model of how the filter will perform. This allows customers to test the model’s signal-to-noise (S/N) performance within their detector based on actual filter performance, rather than estimates.
We can encrypt the coding design files into programs like Zemax or Code V, which has proven extremely useful for our customers. Often, we’ll send a spectral curve representing the spectral shape of an idealized filter, but how is the actual multilayer filter stack going to perform in the customer’s optical chain once the stack is impacted by the optical setup’s angles and other design elements? Optical design software helps customers combine different optical elements and understand different aspects of the system’s performance such as stray light analysis. This cannot be accomplished based only on a spectral curve.
Finally, we are able to work within short lead times. A custom build can take, depending on our customer workload, 16 to 20 weeks for a flight model-appropriate, multi-zone build. In some cases, if a customer only seeks a demonstrator or a proof of principle, leftover materials that are close to their specifications may be used to produce an early benchtop demo.
Built for the Rigors of Space
Optical filters for EO systems must survive the vibration of launch, as well as perform optimally in vacuum and radiation environments. In some cases, satellites are designed to deorbit within three to five years, and the designers/operators require high demisability of components. Namely, they want the component to burn up during atmospheric reentry to minimize the debris falling back to Earth.
Vacuum is not a problem; the filters all are made within a very high vacuum so performance in these conditions is a given. Much like angle of incidence, vibration testing should cover the entirety of the system. Shaking an optic by itself typically will not affect it. However, depending on how the optic is mounted within the system, shaking/twisting/torquing could impact or damage the overall unit and the optical component makers can not anticipate or test for those stresses otherwise.
Regarding the coating technologies used on the optical filters, the sputtering and physical vapor deposition approach we use to make them leads to robust components. Adhesion to the substrates also is strong, and this is by process control and design. We have operated for a long time in the terrestrial telecom field, where optical filter coatings must endure high and low temperature extremes, as well as high humidity, over 25-year+ operational lives.
Our robustness and performance standards do not change whether a satellite system is intended for decades of geostationary orbit or just a few years in low Earth orbit (LEO), though customer expectations for testing tend to be modeled on legacy space agency rules. In some cases, this is prudent: for example, in the past, Iridian Spectral Technologies supported the European Space Agency (ESA) in creating a lightning imager that utilizes a set of two filters, a solar radiation window (SRW) and a narrow bandpass filter (NBF), looking at a single oxygen triplet that is indicative of lightning.6 Those filters were tested very thoroughly to ensure they will function properly for decades, given that there would not be a chance to correct post-launch issues.
Conversely, LEO systems that are designed to deorbit in three to five years do not need to last as long. Ordering a bevy of tests based on historical perceptions of what is needed to work in space can scuttle a project’s cost effectiveness. The ESA’s filter project could comprise a seven-figure price range for a single component, but it makes sense in that context. Ordering components for hundreds of devices, a four-figure price range per component is more viable, especially when the opportunity to adjust and modify performance on subsequent launches is an inherent “continuous improvement” aspect of many of these commercial programs.
OE Observation is Affordable, Accessible, and Life-Saving
Early remote detection of wildfires can support rapid mediation, reducing damage and/or facilitating evacuation where needed. An EO satellite constellation, depending how it is equipped and how many satellites it includes, can allow for updates anywhere between twice a day and every few minutes. In addition to bolstering refresh time through a larger constellation, these systems can be made more effective by improving the size of the imaging and experimenting with optical filter combinations to focus on spectral phenomena of interest. Moreover, engaging partners early enables component suppliers to provide more meaningful data and other contributions to overall system design.
Having supported space agencies and commercial partners around the globe, Iridian Spectral Technologies products have unmatched space heritage — a track record of high-performance components excelling in the most hostile conditions imaginable. We can help customers achieve their EO goals, regardless of the satellite’s orbit, cost, function, or resolution. To learn more, visit https://www.iridian.ca
References:
- “National Interagency Coordination Center Wildland Fire Summary and Statistics Annual Report 2024.” National Interagency Fire Center, 2025. https://www.nifc.gov/sites/default/files/NICC/2-Predictive%20Services/Intelligence/Annual%20Reports/2024/annual_report_2024.pdf
- https://www.msn.com/en-us/news/politics/california-governor-asks-congress-for-nearly-40-billion-for-los-angeles-wildfire-relief/
- Thompson, M. “There Were Over 260 Orbital Launches in 2024. A New Record.” Universe Today, 5 Jan., 2025. https://www.universetoday.com/170295/there-were-over-260-orbital-launches-in-2024-a-new-record/
- CSIS Aerospace Security Project (2022) – processed by Our World in Data. “Launch cost per kilogram of payload” [dataset]. CSIS Aerospace Security Project (2022) [original data]. https://ourworldindata.org/grapher/cost-space-launches-low-earth-orbit
- Kim, J. “SpaceX Launches 131 Payloads to LEO in its 12th Rideshare Mission.” Via Satellite, 14 Jan., 2025. https://www.satellitetoday.com/launch/2025/01/14/spacex-launches-131-payloads-to-leo-in-its-12th-rideshare-mission/
- Montcalm, C. et al. “Solar rejection window and narrow band pass filters for the Meteosat third generation lighting imager”, Proc. SPIE 11180, International Conference on Space Optics — ICSO 2018, 111804Z (12 July 2019); https://doi.org/10.1117/12.2536098